انثى من زمن النقاء


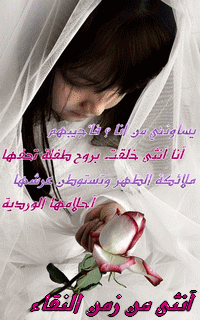
عدد المساهمات : 20012
نقاط الامتيـــــاز : 99165
تاريخ التسجيـل : 10/04/2009 تاريخ الميلاد : 12/06/1973
الوظيفــــــة : 
الهوايـــــــة : 
الجنسيــــــة : 
الدولـــــــة : 
المـــــــزاج : 
جنس العضـو : 
احترام قوانين المنتدى : 
رسالة SMS :
وسائط MMS : 
اوسمة الامتياز : 
اضافات منتديات جسر المحبة توقيت دول العالم: عداد زوار منتديات جسر المحبة: 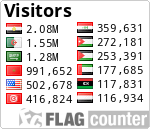
 | موضوع: ترانزستور الوصلة ثنائي القطبية الإثنين فبراير 22, 2010 11:21 am | |
| ترانزستور الوصلة ثنائي القطبية Bipolar Junction Transistor BJT
المحتويات:
فكرة عامة عن تاريخ الترانزستور
مقدمة باللغة العربية عن ترانزستورات الوصلة ثنائية القطبية
شرح مفصّل باللغة الإنجليزية عن:
Bipolar Transistor Basics
The NPN Transistor
The PNP Transistor
Transistor as a Switch
الترانزستورات Transistors تم التغلب على جميع عيوب الصمام الإلكتروني باختراع الترانزستور في عام 1947م وذلك على يد ثلاثة من الفيزيائيين الأميركيين العاملين في مختبرات بيل الأمريكية وهم جون باردين (John Bardeen) و ولتر براتين (Walter Brattain) و وليم شوكلى (William Shockley) والذين حصلوا على جائزة نوبل في عام 1956م تقديرا لجهودهم على هذا الإنجاز العظيم. والترانزستور عنصر إلكتروني فعال (activedevice) مصنوع من مواد شبه موصلة كالجرمانيوم والسيليكون وله ثلاثة أقطاب كما هو الحال مع الصمام الثلاثي ولكن بدون دائرة تسخين. [center] [ندعوك للتسجيل في المنتدى أو التعريف بنفسك لمعاينة هذه الصورة]
ويتميز الترانزستور على الصمام الإلكتروني بصغر حجمه الذي لا يتجاوز إذا ما صنع منفردا حجم حبة الحمص أما إذا كان في دوائر متكاملة فإنه بالإمكان تصنيع ملايين الترانزستورات على شريحة لا تتجاوز مساحتها السنتيمتر المربع الواحد مما أدى إلى تقليص بالغ في أحجام وأوزان الأجهزة الكهربائية.
ويتميز كذلك بأنه يعمل على جهد كهربائي منخفض لا يتجاوز عدة فولتات وبقلة استهلاكه للطاقة الكهربائية التي تقاس بالميللي واط في الترانزستورات المنفردة والميكروواط وحتى النانوواط في الدوائر المتكاملة مما أدى إلى تصنيع أجهزة كهربائية مختلفة تعمل بالبطاريات الصغيرة ولفترات طويلة من الزمن.
ويتميز بصلادته فهو جسم مصمت من مواد شبه موصلة حيث لا توجد في داخله أجزاء متحركة ولذلك فهو لا يتأثر بالصدمات والإهتزازات الميكانيكية كما هو الحال مع الصمام الإلكتروني ولذا يمكن وضعه في الأجهزة المحمولة. ويتميز كذلك بطول عمره التشغيلي الذي يمتد لعشرات السنوات وبإمكانية عمله على نطاق واسع من درجات الحرارة وبإمكانية إنتاجه بكميات كبيرة جدا وبأسعار منخفضة جدا.
ومع اختراع الترانزستور الذي يعده العلماء أعظم اختراع في القرن العشرين تجددت أمال المهندسين في صنع معدات وأجهزة إلكترونية صغيرة الحجم وقليلة الاستهلاك للطاقة كالحواسيب الرقمية والتلفزيونات الملونة والراديوات الصغيرة والهواتف المحمولة والآلات الحاسبة المكتبية واليدوية. وكذلك فإنه لا يوجد الآن ما يحول دون وضع المعدات والأجهزة الإلكترونية في مختلف أنواع المركبات والصواريخ العابرة للقارات وفي المركبات الفضائية والأقمار الصناعية حيث أنها لا تحتل حيزا كبيرا ويمكنها أن تعمل على البطاريات.
ترانزستورات الوصلة ثنائية القطبية Bipolar Junction Transistors (BJT)
يتم تصنيع هذا النوع من الترانزستورات من خلال تطعيم ثلاث مناطق متجاورة على بلورة نقية من السيليكون بحيث يكون التطعيم إما على شكل (سالب_موجب_ سالب)(NPN) أو على شكل (موجب_سالب_موجب) (PNP).
ويتم توصيل أقطاب معدنية بهذه المناطق الثلاثة حيث يسمى القطب الموصول بالمنطقة الوسطى بالقاعدة (****) بينما تسمى الأقطاب الموصولة بالمنطقتين الخارجيتين بالباعث (Emitter) والمجمع (Collector).
ويطلق على هذه النوع من الترانزستورات بالترانزستور ثنائي القطبية (bipolar) وذلك بسبب وجود وصلتين فيه وكذلك بسبب مساهمة الفجوات والإلكترونات في حمل التيار الذي يسري داخل الترانزستور.
يتطلب عمل هذا النوع من الترانزستورات وجود وصلتين يكون في الغالب وضع الإنحياز لأحدهما أمامي وللأخرى عكسي مما يعني أن الوصلة المنحازة أماميا ستسمح بمرور التيار بينما لا تسمح الوصلة المنحازة عكسيا بمروره. ولكن إذا ما تم تصنيع الترانزستور بحيث يكون عرض منطقة القاعدة قليل جدا بحيث أن المنطقة المنضبة للوصلة المنحازة عكسيا تغطي معظم أجزائها فإن الإلكترونات أو الفجوات التي تصل إلى منطقة القاعدة من تيار الوصلة المنحازة أماميا سيقع في أسر المجال الكهربائي للمنطقة المنضبة المنحازة عكسيا وسيمر تيارا عاليا فيها رغم أنها منحازة عكسيا. وكلما قل عرض منطقة القاعدة كلما زادت نسبة عدد الإلكترونات أو الفجوات التي يتم اقتناصها من قبل الوصلة المنحازة عكسيا من العدد الكلي المتولد في الوصلة المنحازة أماميا.
إن هذه الآلية في طريقة عمل الترانزستور تمكن تيارا ضعيفا يمر في القاعدة من التحكم بتيار قوي يمر بين الباعث والمجمع ويطلق على نسبة تيار المجمع أو الباعث على تيار القاعدة بكسب الترانزستور (transistor gain).
ويمكن زيادة كسب الترانزستور من خلال تقليل عرض منطقة القاعدة ويمكن الحصول على كسب قد يصل لعدة مئات. إن العيب الرئيسي للترانزستور ثنائي القطبية هو أن القاعدة تستخدم التيار الكهربائي للتحكم بعمل الترانزستور مما يستدعي استخدام دائرة كهربائية خارجية دقيقة لضبط قيمة تيار القاعدة والذي قد يؤدي أي انحراف في قيمته إلى تغيير مكان نقطة التشغيل التي يعمل عندها الترانزستور.
إن الترانزستورات من نوع (NPN) أكثر شيوعا في الاستخدام من الترانزستورات من نوع (PNP) وذلك لاستجابتها العالية وذلك بسبب أن سرعة حركة الإلكترونات في المناطق السالبة أعلى بكثير من سرعة حركة الفجوات في المناطق الموجبة.
ويتم تصنيع الترانزستور من نوع (NPN) بالطريقة السطحية من خلال تطعيم منطقة محددة بذارات مانحة لتنتج منطقة سالبة بعمق معين على سطح شذرة من السيليكون وفي داخل هذه المنطقة السالبة يتم تطعيم جزء منها بذرات مستقبلة لتحولها إلى منطقة موجبة وفي داخل هذه المنطقة الموجبة يتم تطعيم جزء منها بذرات مانحة لتحولها إلى منطقة سالبة وبهذا تتكون ثلاث مناطق منطقتين سالبتين بينهما منطقة موجبة ويتم وصل القاعدة بالمنطقة الموجبة والباعث بالمنطقة السالبة الأقرب من السطح والمجمع بالمنطقة السالبة الأبعد عن السطح.
وبسبب أن مساحة وصلة الباعث أقل منها بكثير من مساحة وصلة المجمع في عملية التصنيع هذه فإن الكسب في تيار الباعث منه أكبر بكثير منه في حالة تيار المجمع ولذا يجب أن يراعى ذلك عند تصميم المضخمات فالترانزستور غير متماثل في مثل هذه الطريقة من التصنيع.
ويتم تصنيع أنواع لا حصر لها من الترانزستورات بعضها يعمل عند الترددات المنخفضة وبعضها عند الترددات العالية وبعضها لأغراض القدرات المنخفضة وبعضها للقدرات العالية وذلك لتلبي حاجة التطبيقات المختلفة.
ويحمل كل ترانزستور على سطحه رمزا مكون من عدد من الأحرف والأرقام ويمكن استخلاص بعض المعلومات من هذه الرموز كنوع مادة الترانزستور إن كانت من السيليكون أو الجرمانيوم أو كمدى الترددات التي يعمل عندها ومقدار الجهد أو التيار أو القدرة الكهربائية التي يتحملها.
[size=12]Bipolar Transistor
Bipolar Transistor Basics If we now join together two individual diodes end to end giving two PN-junctions connected together in series, we now have a three layer, two junction, three terminal device forming the basis of a Bipolar Junction Transistor, or BJT for short. This type of transistor is generally known as a Bipolar Transistor, because its basic construction consists of two PN-junctions with each terminal or connection being given a name to identify it and these are known as the Emitter, **** and Collector respectively.
The word Transistor is an acronym, and is a combination of the words Transfer Varistor used to describe their mode of operation way back in their early days of development. There are two basic types of bipolar transistor construction, NPN and PNP, which basically describes the physical arrangement of the P-type and N-type semiconductor materials from which they are made. Bipolar Transistors are "CURRENT" Amplifying or current regulating devices that control the amount of current flowing through them in proportion to the amount of biasing current applied to their **** terminal. The principle of operation of the two transistor types NPN and PNP, is exactly the same the only difference being in the biasing (**** current) and the polarity of the power supply for each type.
Bipolar Transistor Construction
The construction and circuit symbols for both the NPN and PNP bipolar transistor are shown above with the arrow in the circuit symbol always showing the direction of conventional current flow between the **** terminal and its emitter terminal, with the direction of the arrow pointing from the positive P-type region to the negative N-type region, exactly the same as for the standard diode symbol.
There are basically three possible ways to connect a Bipolar Transistor within an electronic circuit with each method of connection responding differently to its input signal as the static characteristics of the transistor vary with each circuit arrangement.
• 1. Common **** Configuration - has Vol***e Gain but no Current Gain. • 2. Common Emitter Configuration - has both Current and Vol***e Gain. • 3. Common Collector Configuration - has Current Gain but no Vol***e Gain.
The Common **** Configuration As its name suggests, in the Common **** or Grounded **** configuration, the **** connection is common to both the input signal AND the output signal with the input signal being applied between the **** and the emitter terminals.
The corresponding output signal is taken from between the **** and the collector terminals as shown with the **** terminal grounded or connected to a fixed reference vol***e point. The input current flowing into the emitter is quite large as its the sum of both the **** current and collector current respectively therefore, the collector current output is less than the emitter current input resulting in a Current Gain for this type of circuit of less than "1", or in other words it "Attenuates" the signal.
The Common **** Amplifier Circuit
This type of amplifier configuration is a non-inverting vol***e amplifier circuit, in that the signal vol***es Vin and Vout are In-Phase. This type of arrangement is not very common due to its unusually high vol***e gain characteristics. Its Output characteristics represent that of a forward biased diode while the Input characteristics represent that of an illuminated photo-diode.
Also this type of configuration has a high ratio of Output to Input resistance or more importantly "Load" resistance (RL) to "Input" resistance (Rin) giving it a value of "Resistance Gain". Then the Vol***e Gain for a common **** can therefore be given as:
Common **** Vol***e Gain
The Common **** circuit is generally only used in single s***e amplifier circuits such as microphone pre-amplifier or RF radio amplifiers due to its very good high frequency response.
The Common Emitter Configuration In the Common Emitter or Grounded Emitter configuration, the input signal is applied between the ****, while the output is taken from between the collector and the emitter as shown.
This type of configuration is the most commonly used circuit for transistor ****d amplifiers and which represents the "normal" method of connection. The common emitter amplifier configuration produces the highest current and power gain of all the three bipolar transistor configurations.
This is mainly because the input impedance is LOW as it is connected to a forward-biased junction, while the output impedance is HIGH as it is taken from a reverse-biased junction.
The Common Emitter Amplifier Circuit
In this type of configuration, the current flowing out of the transistor must be equal to the currents flowing into the transistor as the emitter current is given as Ie = Ic + Ib. Also, as the load resistance (RL) is connected in series with the collector, the Current gain of the Common Emitter Transistor Amplifier is quite large as it is the ratio of Ic/Ib and is given the symbol of Beta, (β).
Since the relationship between these three currents is determined by the transistor itself, any small change in the **** current will result in a large change in the collector current.
Then, small changes in **** current will thus control the current in the Emitter/Collector circuit.
By combining the expressions for both Alpha, α and Beta, β the mathematical relationship between these parameters and therefore the current gain of the amplifier can be given as:
Where: "Ic" is the current flowing into the collector terminal, "Ib" is the current flowing into the **** terminal and "Ie" is the current flowing out of the emitter terminal.
Then to summarise, this type of bipolar transistor configuration has a greater input impedance, Current and Power gain than that of the common **** configuration but its Vol***e gain is much lower. The common emitter is an inverting amplifier circuit resulting in the output signal being 180o out of phase with the input vol***e signal.
The Common Collector Configuration In the Common Collector or Grounded Collector configuration, the collector is now common and the input signal is connected to the ****, while the output is taken from the Emitter load as shown. This type of configuration is commonly known as a Vol***e Follower or Emitter Follower circuit. The Emitter follower configuration is very useful for impedance matching applications because of the very high input impedance, in the region of hundreds of thousands of Ohms, and it has relatively low output impedance.
The Common Collector Amplifier Circuit
The Common Emitter configuration has a current gain equal to the β value of the transistor itself. In the common collector configuration the load resistance is situated in series with the emitter so its current is equal to that of the emitter current.
As the emitter current is the combination of the collector AND **** currents combined, the load resistance in this type of amplifier configuration also has both the collector current and the input current of the **** flowing through it. Then the current gain of the circuit is given as:
This type of bipolar transistor configuration is a non-inverting amplifier circuit in that the signal vol***es of Vin and Vout are "In-Phase". It has a vol***e gain that is always less than "1" (unity). The load resistance of the common collector amplifier configuration receives both the **** and collector currents giving a large current gain (as with the Common Emitter configuration) therefore, providing good current amplification with very little vol***e gain.
Bipolar Transistor Summary The behaviour of the bipolar transistor in each one of the above circuit configurations is very different and produces different circuit characteristics with regards to Input impedance, Output impedance and Gain and this is summarised in the table below.
Transistor Characteristics The static characteristics for Bipolar Transistor amplifiers can be divided into the following main groups.
with the characteristics of the different transistor configurations given in the following table:
[/size] The NPN Transistor
In the previous tutorial we saw that the standard Bipolar Transistor or BJT, comes in two basic forms. An NPN (Negative-Positive-Negative) type and a PNP (Positive-Negative-Positive) type, with the most commonly used transistor type being the NPN Transistor. We also learnt that the transistor junctions can be biased in one of three different ways - Common ****, Common Emitter and Common Collector. In this tutorial we will look more closely at the "Common Emitter" configuration using NPN Transistors and an example of its current flow characteristics is given below.
An NPN Transistor Configuration
Note: Conventional current flow.
We know that the transistor is a "CURRENT" operated device and that a large current (Ic) flows freely through the device between the collector and the emitter terminals. However, this only happens when a small biasing current (Ib) is flowing into the **** terminal of the transistor thus allowing the **** to act as a sort of current control input. The ratio of these two currents (Ic/Ib) is called the DC Current Gain of the device and is given the symbol of hfe or nowadays Beta, (β). Beta has no units as it is a ratio. Also, the current gain from the emitter to the collector terminal, Ic/Ie, is called Alpha, (α), and is a function of the transistor itself. As the emitter current Ie is the product of a very small **** current to a very large collector current the value of this parameter α is very close to unity, and for a typical low-power signal transistor this value ranges from about 0.950 to 0.999.
α and β Relationships
By combining the two parameters α and β we can produce two mathematical expressions that gives the relationship between the different currents flowing in the transistor.
The values of Beta vary from about 20 for high current power transistors to well over 1000 for high frequency low power type bipolar transistors. The equation for Beta can also be re-arranged to make Ic as the subject, and with zero **** current (Ib = 0) the resultant collector current Ic will also be zero, (β x 0). Also when the **** current is high the corresponding collector current will also be high resulting in the **** current controlling the collector current. One of the most important properties of the Bipolar Junction Transistor is that a small **** current can control a much larger collector current. Consider the following example.
Example No1.
An NPN Transistor has a DC current gain, (Beta) value of 200. Calculate the **** current Ib required to switch a resistive load of 4mA. Therefore, β = 200, Ic = 4mA and Ib = 20µA.
One other point to remember about NPN Transistors. The collector vol***e, (Vc) must be greater than the emitter vol***e, (Ve) to allow current to flow through the device between the collector-emitter junction. Also, there is a vol***e drop between the **** and the emitter terminal of about 0.7v for silicon devices as the input characteristics of an NPN Transistor are of a forward biased diode. Then the **** vol***e, (Vbe) of an NPN Transistor must be greater than this 0.7 V otherwise the transistor will not conduct with the **** current given as. Where: Ib is the **** current, Vb is the **** bias vol***e, Vbe is the ****-emitter volt drop (0.7v) and Rb is the **** input resistor.
Example No2. An NPN Transistor has a DC **** bias vol***e, Vb of 10v and an input **** resistor, Rb of 100kΩ. What will be the value of the **** current into the transistor. Therefore, Ib = 93µA.
The Common Emitter Configuration. As well as being used as a switch to turn load currents "ON" or "OFF" by controlling the **** signal to the transistor, NPN Transistors can also be used to produce a circuit which will also amplify any small AC signal applied to its **** terminal. If a suitable DC "biasing" vol***e is firstly applied to the transistors **** terminal thus allowing it to always operate within its linear active region, an inverting amplifier circuit called a Common Emitter Amplifier is produced. One such Common Emitter Amplifier configuration is called a Class A Amplifier. A Class A Amplifier operation is one where the transistors **** terminal is biased in such a way that the transistor is always operating halfway between its cut-off and saturation points, thereby allowing the transistor amplifier to accurately reproduce the positive and negative halves of the AC input signal superimposed upon the DC Biasing vol***e. Without this "Bias Vol***e" only the positive half of the input waveform would be amplified. This type of amplifier has many applications but is commonly used in audio circuits such as pre-amplifier and power amplifier s***es. With reference to the common emitter configuration shown below, a family of curves known commonly as the Output Characteristics Curves, relates the output collector current, (Ic) to the collector vol***e, (Vce) when different values of **** current, (Ib) are applied to the transistor for transistors with the same β value. A DC "Load Line" can also be drawn onto the output characteristics curves to show all the possible operating points when different values of **** current are applied. It is necessary to set the initial value of Vce correctly to allow the output vol***e to vary both up and down when amplifying AC input signals and this is called setting the operating point or Quiescent Point, Q-point for short and this is shown below.
The Common Emitter Amplifier Circuit
Output Characteristics Curves for a Typical Bipolar Transistor
The most important factor to notice is the effect of Vce upon the collector current Ic when Vce is greater than about 1.0 volts. You can see that Ic is largely unaffected by changes in Vce above this value and instead it is almost entirely controlled by the **** current, Ib. When this happens we can say then that the output circuit represents that of a "Constant Current Source". It can also be seen from the common emitter circuit above that the emitter current Ie is the sum of the collector current, Ic and the **** current, Ib, added together so we can also say that " Ie = Ic + Ib " for the common emitter configuration. By using the output characteristics curves in our example above and also Ohm´s Law, the current flowing through the load resistor, (RL), is equal to the collector current, Ic entering the transistor which inturn corresponds to the supply vol***e, (Vcc) minus the vol***e drop between the collector and the emitter terminals, (Vce) and is given as:
Also, a Load Line can be drawn directly onto the graph of curves above from the point of "Saturation" when Vce = 0 to the point of "Cut-off" when Ic = 0 giving us the "Operating" or Q-point of the transistor. These two points are calculated as:
Then, the collector or output characteristics curves for Common Emitter NPN Transistors can be used to predict the Collector current, Ic, when given Vce and the **** current, Ib. A Load Line can also be constructed onto the curves to determine a suitable Operating or Q-point which can be set by adjustment of the **** current.
The PNP Transistor
The PNP Transistor is the exact opposite to the NPN Transistor device we looked at in the previous tutorial. Basically, in this type of transistor construction the two diodes are reversed with respect to the NPN type, with the arrow, which also defines the Emitter terminal this time pointing inwards in the transistor symbol. Also, all the polarities are reversed which means that PNP Transistors "sink" current as opposed to the NPN transistor which "sources" current. Then, PNP Transistors use a small output **** current and a negative **** vol***e to control a much larger emitter-collector current. The construction of a PNP transistor consists of two P-type semiconductor materials either side of the N-type material as shown below.
A PNP Transistor Configuration
Note: Conventional current flow.
The PNP Transistor has very similar characteristics to their NPN bipolar cousins, except that the polarities (or biasing) of the current and vol***e directions are reversed for any one of the possible three configurations looked at in the first tutorial, Common ****, Common Emitter and Common Collector. Generally, PNP Transistors require a negative (-ve) vol***e at their Collector terminal with the flow of current through the emitter-collector terminals being Holes as opposed to Electrons for the NPN types. Because the movement of holes across the depletion layer tends to be slower than for electrons, PNP transistors are generally more slower than their *****alent NPN counterparts when operating. To cause the **** current to flow in a PNP transistor the **** needs to be more negative than the Emitter (current must leave the ****) by approx 0.7 volts for a silicon device or 0.3 volts for a germanium device with the formulas used to calculate the **** resistor, **** current or Collector current are the same as those used for an *****alent NPN transistor and is given as.
[size=21]Generally, the PNP transistor can replace NPN transistors in electronic circuits, the only difference is the polarities of the vol***es, and the directions of the current flow. PNP Transistors can also be used as switching devices and an example of a PNP transistor switch is shown below.
A PNP Transistor Circuit
The Output Characteristics Curves for a PNP transistor look very similar to those for an *****alent NPN transistor except that they are rotated by 180o to take account of the reverse polarity vol***es and currents, (the currents flowing out of the **** and Collector in a PNP transistor are negative).
Transistor Matching You may think what is the point of having a PNP Transistor, when there are plenty of NPN Transistors available?. Well, having two different types of transistors PNP & NPN, can be an advan***e when designing amplifier circuits such as Class B Amplifiers that use "Complementary" or "Matched Pair" transistors or for reversible H-Bridge motor control circuits. A pair of corresponding NPN and PNP transistors with near identical characteristics to each other are called Complementary Transistors for example, a TIP3055 (NPN), TIP2955 (PNP) are good examples of complementary or matched pair silicon power transistors. They have a DC current gain, Beta, (Ic / Ib) matched to within 10% and high Collector current of about 15A making them suitable for general motor control or robotic applications.
Identifying the PNP Transistor We saw in the first tutorial of this Transistors section, that transistors are basically made up of two Diodes connected together back-to-back. We can use this analogy to determine whether a transistor is of the type PNP or NPN by testing its Resistance between the three different leads, Emitter, **** and Collector. By testing each pair of transistor leads in both directions will result in six tests in total with the expected resistance values in Ohm's given below.
• 1. Emitter-**** Terminals - The Emitter to **** should act like a normal diode and conduct one way only. • 2. Collector-**** Terminals - The Collector-**** junction should act like a normal diode and conduct one way only. • 3. Emitter-Collector Terminals - The Emitter-Collector should not conduct in either direction.
Transistor Resistance Values for the PNP transistor and NPN transistor types
[ندعوك للتسجيل في المنتدى أو التعريف بنفسك لمعاينة هذه الصورة]. [size=12]The Transistor as a Switch
When used as an AC signal amplifier, the transistors **** biasing vol***e is applied so that it operates within its "Active" region and the linear part of the output characteristics curves are used. However, both the NPN & PNP type bipolar transistors can be made to operate as an "ON/OFF" type solid state switch for controlling high power devices such as motors, solenoids or lamps. If the circuit uses the Transistor as a Switch, then the biasing is arranged to operate in the output characteristics curves seen previously in the areas known as the "Saturation" and "Cut-off" regions as shown below.
Transistor Curves [center] [ندعوك للتسجيل في المنتدى أو التعريف بنفسك لمعاينة هذه الصورة]
The pink shaded area at the bottom represents the "Cut-off" region. Here the operating conditions of the transistor are zero input **** current (Ib), zero output collector current (Ic) and maximum collector vol***e (Vce) which results in a large depletion layer and no current flows through the device. The transistor is switched "Fully-OFF". The lighter blue area to the left represents the "Saturation" region. Here the transistor will be biased so that the maximum amount of **** current is applied, resulting in maximum collector current flow and minimum collector emitter vol***e which results in the depletion layer being as small as possible and maximum current flows through the device. The transistor is switched "Fully-ON". Then we can summarize this as:
• 1. Cut-off Region - Both junctions are Reverse-biased, **** current is zero or very small resulting in zero Collector current flowing, the device is switched fully "OFF". • 2. Saturation Region - Both junctions are Forward-biased, **** current is high enough to give a Collector-Emitter vol***e of 0v resulting in maximum Collector current flowing, the device is switched fully "ON".
An example of an NPN Transistor as a switch being used to operate a relay is given below. With inductive loads such as relays or solenoids a flywheel diode is placed across the load to dissipate the back EMF generated by the inductive load when the transistor switches "OFF" and so protect the transistor from damage. If the load is of a very high current or vol***e nature, such as motors, heaters etc, then the load current can be controlled via a suitable relay as shown.
Transistor Switching Circuit
The circuit resembles that of the Common Emitter circuit we looked at in the previous tutorials. The difference this time is that to operate the transistor as a switch the transistor needs to be turned either fully "OFF" (Cut-off) or fully "ON" (Saturated). An ideal transistor switch would have an infinite resistance when turned "OFF" resulting in zero current flow and zero resistance when turned "ON", resulting in maximum current flow. In practice when turned "OFF", small leakage currents flow through the transistor and when fully "ON" the device has a low resistance value causing a small saturation vol***e (Vce) across it. In both the Cut-off and Saturation regions the power dissipated by the transistor is at its minimum.
To make the **** current flow, the **** input terminal must be made more positive than the Emitter by increasing it above the 0.7 volts needed for a silicon device. By varying the ****-Emitter vol***e Vbe, the **** current is altered and which in turn controls the amount of Collector current flowing through the transistor as previously discussed. When maximum Collector current flows the transistor is said to be Saturated. The value of the **** resistor determines how much input vol***e is required and corresponding **** current to switch the transistor fully "ON".
Example No1. For example, using the transistor values from the previous tutorials of: β = 200, Ic = 4mA and Ib = 20uA, find the value of the **** resistor (Rb) required to switch the load "ON" when the input terminal vol***e exceeds 2.5v.
Example No2. Again using the same values, find the minimum **** current required to turn the transistor fully "ON" (Saturated) for a load that requires 200mA of current.
Transistor switches are used for a wide variety of applications such as interfacing large current or high vol***e devices like motors, relays or lamps to low vol***e digital logic IC's or gates like AND Gates or OR Gates. Here, the output from a digital logic gate is only +5v but the device to be controlled may require a 12 or even 24 volts supply. Or the load such as a DC Motor may need to have its speed controlled using a series of pulses (Pulse Width Modulation) and transistor switches will allow us to do this faster and more easily than with conventional mechanical switches.
Digital Logic Transistor Switch
The **** resistor, Rb is required to limit the output current of the logic gate.
Darlington Transistors Sometimes the DC current gain of the bipolar transistor is too low to directly switch the load current or vol***e, so multiple switching transistors are used. Here, one small input transistor is used to switch "ON" or "OFF" a much larger current handling output transistor. To maximise the signal gain the two transistors are connected in a "Complementary Gain Compounding Configuration" or what is generally called a "Darlington Configuration" where the amplification factor is the product of the two individual transistors. Darlington Transistors simply contain two individual bipolar NPN or PNP type transistors connected together so that the current gain of the first transistor is multiplied with that of the current gain of the second transistor to produce a device which acts like a single transistor with a very high current gain. The overall current gain Beta (β) or Hfe value of a Darlington device is the product of the two individual gains of the transistors and is given as:
So Darlington Transistors with very high β values and high Collector currents are possible compared to a single transistor. An example of the two basic types of Darlington transistor are given below.
Darlington Transistor Configurations
The above NPN Darlington transistor configuration shows the Collectors of the two transistors connected together with the Emitter of the first transistor connected to the **** of the second transistor therefore, the Emitter current of the first transistor becomes the **** current of the second transistor.
The first or "input" transistor receives an input signal, amplifies it and uses it to drive the second or "output" transistors which amplifies it again resulting in a very high current gain. As well as its high increased current and vol***e switching capabilities, another advan***e of a Darlington transistor is in its high switching speeds making them ideal for use in Inverter circuits and DC motor or stepper motor control applications. One difference to consider when using Darlington transistors over the conventional single bipolar transistor type is that the ****-Emitter input vol***e Vbe needs to be higher at approx 1.4v for silicon devices, due to the series connection of the two PN junctions.
Then to summarise when using a Transistor as a Switch
• Transistor switches can be used to switch and control lamps, relays or even motors. • When using bipolar transistors as switches they must be fully "OFF" or fully "ON". • Transistors that are fully "ON" are said to be in their Saturation region. • Transistors that are fully "OFF" are said to be in their Cut-off region. • In a transistor switch a small **** current controls a much larger Collector current. • When using transistors to switch inductive relay loads a "Flywheel Diode" is required. • When large currents or vol***es need to be controlled, Darlington Transistors are used
[/size][/center]
[/size][/center] | |
|